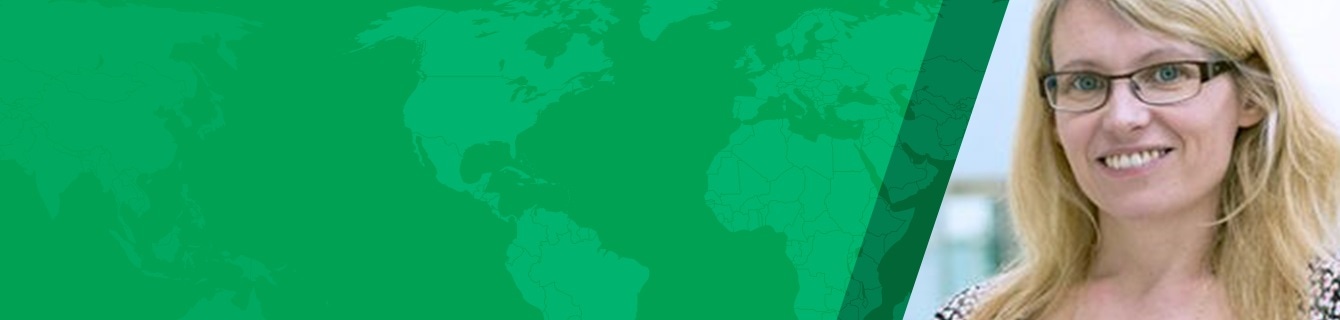
An interview with Prof. Aline Miller, BSc(Hons) PhD FRSC FInstP, Chief Executive Office, Director and Founder, Manchester BIOGEL
Please give an overview of organoids. Why are they an important research model?
Organoids are 3-dimensional (3D) clusters of stem cells that come together and emulate the microenvironment within individual organs, whether that be liver, kidney, heart, gut or other specific organs. Essentially, they can be viewed as miniature, simplified organs. They typically range in size from a few micrometers to five millimeter and there are potentially as many different organoids as there are different tissues and organs in the body. Such a diverse range of organoids can form by controlling the differentiation of the specific stem cell used, which can be influenced by the cells receiving instructive signals from the 3D extracellular matrix (ECM), the medium and once the 3D structure assembles, by the cells present in the organoids themselves.
Image credit: Dimarion | Shutterstock
There is considerable attention and excitement surrounding organoids at the moment as they have the potential to revolutionize the way diseases are studied and treated. Growing human derived tissues in the tissue culture dish opens up the opportunity to study the fundamental cell biology within individual organs, model human disease from individual patients and test reliably new drug compounds to decrease late stage failure of therapeutics and also in a personalized medicine approach. In addition, there is the longer-term potential of growing whole organs and using them for transplantation, thus replacing the need for organ donations.
How are organoids 3D printed in the lab, particularly high throughput production?
3D printing allows researchers to generate increasingly complex structures of living systems. One application is lab-on-a-chip, alternatively known as organs-on-a-chip. This involves printing and connecting different organoids together to create a 3D human-like pathway. These are typically used for testing the toxicity and efficacy of new drugs as they pass through the organs within the chip, which is aiming to replicate the human body environment. The key aim of this is to increase drug discovery, decrease late stage failure rate of therapeutics, advance personalized medicine and decrease animal testing.
High through-put production involves printing repeating cell structures, or cell containing droplets, that lead to the same organoid forming in multiple arrays. This enables many comparable tests to be carried out at the same time, and in quick succession. The advantage of such a high throughput environment, is you are able to explore quickly the affect of a high number of variables on the system, using very small volumes of sample. It is quicker, cheaper and easier than traditional methods. Recent advances in robotic high throughput liquid handling has expedited this aspect of the work well, in addition to the development of printable hydrogels ‘bioinks’.
How does the high throughput aspect of organoid production affect reliability? Does it change how they interact with the drugs?
Reliability and reproducibility are paramount when printing organoids for drug discovery At the moment, I think that the challenges of achieving this lie with the reliability of what it is exactly that you are printing in the first instance. Are you printing the same number of cells each time? Do all your cells survive the printing process? Can the printed cells cluster in the right way to form the organoids you want to grow? How consistent can the printing process be within one experiment? How consistent can this be between different experiments and different labs?
Each of the above needs to be known and controlled to enable cell survival in the printing process and the growth of organoids of the same size and type, every time. I believe these challenges can be overcome by taking a materials engineering approach. This means using a known, well characterized and consistent hydrogel as the ECM. This will allow the printing parameters (volume, needle size, cell number, printing pressure, speed and time) to be predicted and determined to ensure reproducibly and reliable printing for the formation of consistent organoids. Only then will the specific organ cell biology be replicated to give data that is consistent, reproducible, reliable, efficient and scalable.
What does Manchester BIOGEL offer for 3D organoid printing?
Manchester BIOGEL offers 3D synthetic peptide hydrogel systems, PeptiGels® that are fully printable and reproducible. The hydrogels are fibrillar scaffolds, reminiscent of natural ECM, that essentially provide a climbing frame for the cells to go onto, move around, interact with, differentiate and grow on. The mechanical properties and functionality of these scaffolds are highly tunable to create environments that mimic all human tissues. Importantly our peptide products, PeptiGels® are shear thinning which means they are easily printable and they regain their gel properties immediately after release from the printing needle.
Image credit: Manchester BIOGEL
Such properties allow the stem cells to cluster in a reproducible and reliable way. It also protects the cells and ultimately the organoid growth during the 3D printing process. This protection is important, because when you print you are putting a lot of shear stress onto the cells, and onto the materials. Encapsulation within the hydrogel helps ensure the cells both survive the 3D printing process and retain their ability to form the desired organoid.
Our hydrogels also allow you to print 3D structures with a high spatial resolution. This allows you to do more complex things like print specific microstructures within a larger organ. For example, you can print vascularization within a heart and print different types of cells to replicate different parts of the organ with our tailored PeptiGels®.
Why is the scalability of the Manchester BIOGEL technology beneficial?
Our materials are fully scalable and are manufactured under ISO13486 standards with complete reproducibility – we guarantee no batch to batch variability. Having a 3D material that exhibits these characteristics is crucial for organoid production, for any application.
One other key need is the ability to recreate human tissue safely in develop clinically translatable systems. The material mainly used currently is Matrigel, which is a decellularized tumour grown within mice. This animal derived system is well-known to have huge batch-to-batch variability and also contains a ‘soup’ of growth factors and cell recognition sequences that influences cell behavior in unknown and unpredictable ways. As a consequence, you cannot be sure if the cell behavior you are getting is actually due to the cell response or whether it is due to something present in the Matrigel material. Moreover, any R&D developed using this system will never be translated into the clinic due to it being sourced from animals.
You can remove these risk factors by working with fully synthetic hydrogels, because you know exactly what is in them and you know they do not elicit any specific cell response other than what you dial in.
Why is the extracellular matrix so important when 3D printing organoids? How can the extracellular matrix be manipulated by researchers?
The extracellular matrix (ECM) is critical when 3D printing organoids. It not only offers protection to the cells from the high stresses and strain of the printing process, but also provides matrix support to encourage cell clustering and directed differentiation once printed.
Peptide hydrogels are ideal ECM mimics for bioprinting; they are inherently biocompatible, can be easily manipulated in terms of their mechanical strength and (bio) functionality can easily be introduced to direct differentiation. These are key to ensure human behavior of the organoid can be replicated.
More specifically, the stiffness of the hydrogel can be tuned to match the properties of the in vivo tissue, for example the stiffness of brain tissue is much less than the stiffness of heart tissue. This is achieved partly through changing the concentration and partly through changing the peptide type that we use to formulate our materials. In addition, cell instructive sequences can be embedded within the hydrogel in a systematic and modular fashion providing complete control over cell response and behavior. Typical functional groups that can be incorporated include -RGD (fibronectin), -IKVAV and YIGSR (laminin) and -GFOGER (collagen) allowing us to replicate components that are naturally present within certain environments in vivo.
Then we can also add specific growth factors, sugars or glycosaminoglycans into the system, as desired by our customers.
What types of organoids can be produced? Are there any tissue types and structures that cannot yet be 3D printed?
I believe any tissue type or organ can be reproduced mainly because there are recent advances in controlling the differentiation of stem cells into different lineages and also that the properties of hydrogels can be tuned to replicate any human tissue type.
I am not aware of any tissues that cannot be printed yet. However, there are many still in development in different research groups around the world. Some examples of what we have looked at specifically with our hydrogels are things like liver, kidney and cardiac organoids. We have also done some gastrointestinal organoids and we have had a particular focus on different types of tumor organoids at different stages of disease progression.
Please give an overview of 3D bioprinting in cancer research. Can organoids be printed with tumor cells? Can tumors be printed?
3D bioprinting of cancer models is beginning to emerge as an area of growth. This is driven partly by a need for researchers to move away from 2D cultures, i.e. flat biology and into the 3D space to really replicate in vivo tumor behavior, and partly by the need to print more complex structures, for example represent the vascularization embedded within tumours. Much of this can be achieved by printing multiple cell lines, together or separately, embedded within designed hydrogels to re-create the different regions within tumours.
One interesting recent development is manipulating the properties of the scaffold individually using PeptiGels®. For example, we are able to tune the pH and stiffness independently to replicate the environment of healthy and tumour tissue, as well as tumour tissue at different stages of progression. Excitingly, this has been shown with breast cancer cells and pancreatic cancer cell lines to lead to differences within the cell biology pathways.
How can 3D printed organoids be utilized in personalized medicine?
Printing 3D organoids can help develop personalized medicines in two ways; firstly it can increase the precision of the diagnosis of a condition, and secondly it can help find the right therapeutic, or mixture of drugs, to provide the optimal therapeutic response for individual patients. We are all different, and have unique complex molecular and cellular processes. Consequently, we have vastly different toxicity and efficacy profiles to the same drug/s. For example, currently only 30-60% of patient treatments are effective due to differences in the way an individual responds to, and metabolizes, medicines.
By capitalizing on recent advances in stem cell technology we can now take a sample of a patient’s own stem cells, and combine this with high-throughput technologies to print and culture them into multiple organoid arrays. This will allow us to explore, understand and identify the genetic profiles responsible for individual patients drug response and use it to establish their ‘pharmacogenomic’ profile, and identify optimal treatment.
This will allow us to move away from our current ‘trial and error’ prescribing to optimal therapy for all first time round.
Are there any areas that you think will be heavily impacted by 3D printed organoids in the future?
As previously mentioned, I think the key areas where 3D printing of organoids can create a step change are within the growing fields of drug discovery, personalized medicines, tissue engineering and understanding and enhancing the treatment of diseases, including cancer.
Within each of these areas 3D bioprinting opens up the opportunity to develop a deeper understanding of the underlying science as well as increase the efficacy of therapeutics, reduce their development cost and lead to a paradigm shift in disease treatment.
In summary it will enhance our quality of life.
What do you see as the future of 3D printed organoids and for Manchester BIOGEL?
Manchester BIOGEL is emerging as a market leader in the design and supply of peptide hydrogels, PeptiGels®, as these materials are printable ECM mimics with a proven track record to host a range of different stem cells, and cell differentiation has been directed into multiple different organoids. Examples include, but are not limited to liver, kidney, cardiac, gastrointestinal and tumour systems.
Importantly our PeptiGels can be printed into complex structure to mimic full organs using traditional bioprinting methodologies, while also being able to form micro droplets (down to 2ml) while maintaining call viability and structural integrity using liquid handling techniques.
Overall, we are excited to contribute to this growing area and becoming the key player in enabling more human like behavior being replicated in the lab, reducing the use of animals in drug research and offering clinically translatable solutions. All this in parallel with saving researchers time and resource by enabling the generation of reproducible and reliable results.
Where can readers find more information?
About Prof. Aline Miller, BSc(Hons) PhD FRSC FInstP
Aline currently oversees and leads on all aspects of the business and has over two decades of experience guiding commercial and academic teams across the life science sector. She has a strong track record of raising funds to drive the translation of academic research into the clinical and commercial setting.
Before taking the CEO role, Aline was Professor of Biomolecular Engineering at The University of Manchester. She is a chemist by training and also held a New Hall Junior Research Fellowship at the University of Cambridge.
Sponsored Content Policy: News-Medical.net publishes articles and related content that may be derived from sources where we have existing commercial relationships, provided such content adds value to the core editorial ethos of News-Medical.Net which is to educate and inform site visitors interested in medical research, science, medical devices and treatments.
https://news.google.com/__i/rss/rd/articles/CBMiYmh0dHBzOi8vd3d3Lm5ld3MtbWVkaWNhbC5uZXQvbmV3cy8yMDIwMDgyOC9NYW5pcHVsYXRpbmctM0QtUHJpbnRlZC1Pcmdhbm9pZC1NaWNyb2Vudmlyb25tZW50cy5hc3B40gFmaHR0cHM6Ly93d3cubmV3cy1tZWRpY2FsLm5ldC9hbXAvbmV3cy8yMDIwMDgyOC9NYW5pcHVsYXRpbmctM0QtUHJpbnRlZC1Pcmdhbm9pZC1NaWNyb2Vudmlyb25tZW50cy5hc3B4?oc=5
2020-08-28 09:41:00Z
CAIiENw0e2KaJc6N8SE38pE0wKoqMwgEKioIACIQZdRflS9INK7zM5FkBi3R3CoUCAoiEGXUX5UvSDSu8zORZAYt0dww_7XIBg
Bagikan Berita Ini
0 Response to "Manipulating 3D Printed Organoid Microenvironments - News-Medical.Net"
Post a Comment